Research Page
Cloud Remote Sensing and Modeling
Clouds play a critical role in the Earth's hydrological cycle and in the energy balance of the climate system. They strongly influence solar heating by reflecting part of the incident solar radiation back to space. Clouds also reduce the planet’s ability to cool by intercepting part of the thermal infrared radiation emitted by the surface and atmosphere below, and re-emitting a fraction of this radiation back towards the surface. Global changes in surface temperature are highly sensitive to cloud amount and type, a major reason for the largest uncertainty in model estimates of global warming being due to clouds.
Cloud studies require determination of both macrophysical (e.g., cloud cover) and microphysical (e.g., cloud particle size) cloud properties on horizontal scales ranging from a few tens of meters to hundreds of kilometers, based on modeling and on measurements taken from surface, aircraft and satellite vantage points. Satellites are critically important because they can provide global observational coverage. The CRL is a worldwide leader in implementing operational algorithms that retrieve cloud properties from measurements of prominent satellite sensors used for that role such as MODIS (and its successor variants like VIIRS, ABI, EPIC, etc.). However, remote sensing techniques and climate models almost always employ a “plane-parallel” cloud approximation in their radiation codes, i.e., clouds are assumed to be flat, homogeneous, infinite slabs. Because clouds are in reality quite inhomogeneous, as evidenced by even casual glances at the sky, CRL scientists study the impacts of this unrealistic approximation in a variety of ways and for a variety of applications. CRL is a global leader in computations of reflection, absorption and transmission of highly inhomogeneous clouds with various 3-dimensional radiative transfer techniques, such as Monte Carlo and spherical-harmonic discrete ordinates. Lab scientists have organized and brought to fruition the international Intercomparison of 3D Radiation Codes (I3RC) to determine the efficiency and relative accuracy of these techniques, and are leaders in international efforts such as the International Cloud Working Group (ICWG).
One of the most prominent problems tackled by CRL scientists is how to distinguish cloud from aerosol particles. Despite preconceptions, this distinction is not simply an altitude dependence where cloud-like entities can be found at ground level (e.g., fog and blowing snow) and aerosols can be found in the stratosphere (e.g., from large volcanic eruptions). Nor is it a matter of local particle concentrations: highly opaque aerosol plumes (e.g., from wildfires) can form, and so do invisible to the naked eye (“sub-visible”) cirrus clouds. From the radiation transport perspective, when two extreme regimes co-exist such as when optically thin (cloudless) regions neighbor optically thick (cloudy) regions, observational and modeling challenges arise. CRL radiation scientists study not only the individual regimes, but also their transition zone, a region of strong aerosol-cloud interactions where aerosol particles humidify and swell, while cloud drops evaporate and shrink. Such areas are ubiquitous: according to Cloud-Aerosol Lidar and Infrared Pathfinder Satellite (CALIPSO) observations, about half of all ‘clear’ pixels over ocean are within 5 km of a low cloud. The transition zone complicates estimates of both aerosol effects on clouds (the so-called “indirect effects”), but also of direct aerosol radiative effects: excluding aerosols near clouds would dramatically reduce the available data and would lead to underestimates of their forcing, while including them would lead to overestimates because of unaccounted cloud contamination.
Cloud research within CRL also involves one of our most important tools for predicting climate change -- Global Climate Models (GCMs). GCMs are complex computer models that attempt to simulate physical processes taking place in the Earth's atmosphere, oceans, and land surface. One key limiting factor in the quality of climate predictions by current GCMs is the realistic representation of cloud properties: cloud cover, amount of cloud water, and number and size of droplets or ice crystals that make up clouds. It is important to simulate cloud properties accurately because, as mentioned earlier, clouds largely determine the amount of solar and thermal radiation reaching the surface and being reflected and emitted to space. As GCM realizations of cloud-precipitation processes approach those in mesoscale models, it is also imperative to simulate cloud properties correctly. One aspect of cloud realism that is often overlooked is the way cloud cover and other cloud properties are distributed with height and how they overlap. Lab scientists use vertical cloud structures observed by active sensors (e.g., CloudSat, CALIPSO) and a wide range of cloud property products from a variety of other satellite platforms to validate with sophisticated analysis advanced cloud schemes of large-scale models (including GSFC’s own GEOS model).
Contact: Kerry Meyer
Cloud studies require determination of both macrophysical (e.g., cloud cover) and microphysical (e.g., cloud particle size) cloud properties on horizontal scales ranging from a few tens of meters to hundreds of kilometers, based on modeling and on measurements taken from surface, aircraft and satellite vantage points. Satellites are critically important because they can provide global observational coverage. The CRL is a worldwide leader in implementing operational algorithms that retrieve cloud properties from measurements of prominent satellite sensors used for that role such as MODIS (and its successor variants like VIIRS, ABI, EPIC, etc.). However, remote sensing techniques and climate models almost always employ a “plane-parallel” cloud approximation in their radiation codes, i.e., clouds are assumed to be flat, homogeneous, infinite slabs. Because clouds are in reality quite inhomogeneous, as evidenced by even casual glances at the sky, CRL scientists study the impacts of this unrealistic approximation in a variety of ways and for a variety of applications. CRL is a global leader in computations of reflection, absorption and transmission of highly inhomogeneous clouds with various 3-dimensional radiative transfer techniques, such as Monte Carlo and spherical-harmonic discrete ordinates. Lab scientists have organized and brought to fruition the international Intercomparison of 3D Radiation Codes (I3RC) to determine the efficiency and relative accuracy of these techniques, and are leaders in international efforts such as the International Cloud Working Group (ICWG).
One of the most prominent problems tackled by CRL scientists is how to distinguish cloud from aerosol particles. Despite preconceptions, this distinction is not simply an altitude dependence where cloud-like entities can be found at ground level (e.g., fog and blowing snow) and aerosols can be found in the stratosphere (e.g., from large volcanic eruptions). Nor is it a matter of local particle concentrations: highly opaque aerosol plumes (e.g., from wildfires) can form, and so do invisible to the naked eye (“sub-visible”) cirrus clouds. From the radiation transport perspective, when two extreme regimes co-exist such as when optically thin (cloudless) regions neighbor optically thick (cloudy) regions, observational and modeling challenges arise. CRL radiation scientists study not only the individual regimes, but also their transition zone, a region of strong aerosol-cloud interactions where aerosol particles humidify and swell, while cloud drops evaporate and shrink. Such areas are ubiquitous: according to Cloud-Aerosol Lidar and Infrared Pathfinder Satellite (CALIPSO) observations, about half of all ‘clear’ pixels over ocean are within 5 km of a low cloud. The transition zone complicates estimates of both aerosol effects on clouds (the so-called “indirect effects”), but also of direct aerosol radiative effects: excluding aerosols near clouds would dramatically reduce the available data and would lead to underestimates of their forcing, while including them would lead to overestimates because of unaccounted cloud contamination.
Cloud research within CRL also involves one of our most important tools for predicting climate change -- Global Climate Models (GCMs). GCMs are complex computer models that attempt to simulate physical processes taking place in the Earth's atmosphere, oceans, and land surface. One key limiting factor in the quality of climate predictions by current GCMs is the realistic representation of cloud properties: cloud cover, amount of cloud water, and number and size of droplets or ice crystals that make up clouds. It is important to simulate cloud properties accurately because, as mentioned earlier, clouds largely determine the amount of solar and thermal radiation reaching the surface and being reflected and emitted to space. As GCM realizations of cloud-precipitation processes approach those in mesoscale models, it is also imperative to simulate cloud properties correctly. One aspect of cloud realism that is often overlooked is the way cloud cover and other cloud properties are distributed with height and how they overlap. Lab scientists use vertical cloud structures observed by active sensors (e.g., CloudSat, CALIPSO) and a wide range of cloud property products from a variety of other satellite platforms to validate with sophisticated analysis advanced cloud schemes of large-scale models (including GSFC’s own GEOS model).
Contact: Kerry Meyer
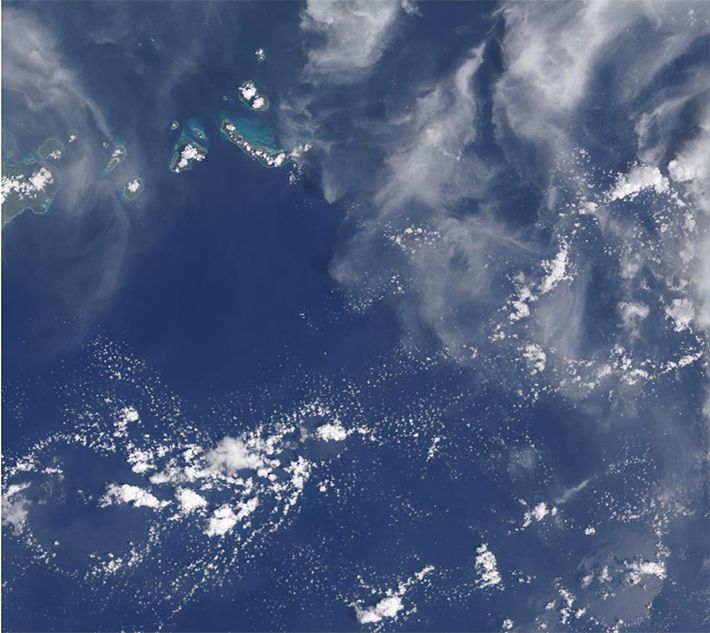